Triton Explains: Acoustic Particle Motion and Marine Energy
Have you ever wondered how fishes and invertebrates hear? Unlike mammals, which rely on changes in sound pressure to hear, fishes and aquatic invertebrates hear by detecting acoustic particle motion as well as substrate vibration.
Research on underwater noise from human activities has historically primarily focused on the impacts of noise on marine mammals. However, fishes and invertebrates play critical roles in ecosystems regarding biodiversity, food web dynamics, and human uses like commercial and subsistence fisheries that feed populations worldwide. According to Triton Principal Investigator and underwater acoustics researcher at Pacific Northwest National Laboratory-Sequim (PNNL-Sequim) Joe Haxel, PhD, “if you want to understand noise effects on fishes and aquatic invertebrates, you have to consider acoustic particle motion and substrate vibration.”
The Department of Energy (DOE) Water Power Technologies Office (WPTO) Triton Initiative aims to understand various environmental stressors associated with marine energy systems and their impacts on receptors—marine animals and their ecosystems. Triton is conducting acoustic particle motion research to address knowledge gaps related to the potential impacts of marine energy-generated underwater noise on fishes and invertebrates to better understand underwater noise effects for all species.
Hearing Using Sound Pressure vs. Particle Motion
Underwater sound is characterized by two interrelated components: sound pressure and particle motion. Sound travels through water (or any medium) in the form of sound pressure waves. Individual water particles that interact with sound waves do not travel with the wave. Instead, the particles vibrate back and forth, as seen in the animation below. This is called particle motion. When these vibrations are generated through sediment, it is known as substrate vibration.
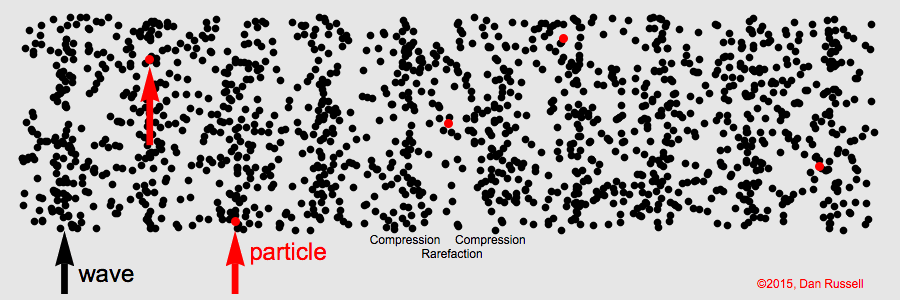
Research has shown that all fishes detect particle motion and that most aquatic invertebrates that detect sound also use particle motion rather than sound pressure (Popper and Hawkins, 2018). In addition to detecting particle motion in the water, sound-detecting aquatic invertebrates and fishes that live near, on, or in the substrate are also likely to use their auditory systems to detect substrate vibration.
Our understanding of fish hearing has benefitted from decades of research by fish behaviorists and physiologists. Fish ears and related structures have been evolving for hundreds of millions of years. The ears in fishes contain thousands, or even tens of thousands, of sensory hair cells—the same type of sensory cells found in our own ears. Each sensory cell in a fish’s ear has a tuft of cilia (tiny, hair-like structures) projecting from its surface. These cilia are in contact with a calcium carbonate structure—in most fishes it is a solid structure called an otolith (or a gelatinous mass of calcium carbonate crystals, called otoconia, in some primitive fishes and in sharks and rays). The bodies of all fishes are roughly the same density as water and generally “move” with the motion of water particles. However, the otolith (or otoconial mass), which is about three times denser than the rest of the body, moves differently relative to surrounding tissues. This difference leads to the bending of the cilia, which stimulates the sensory hair cells and sends signals to the brain through the nervous system. This direct ear stimulation enables fishes to hear and respond to sounds in their environments (Popper and Fay, 2011).
The otolith organs in fish ears act as small accelerometers—which sense and measure the three-dimensional (3-D) vibration, or motion of the water molecules. All fishes (and sharks) sense particle motion, while only some fishes (but no sharks) can also detect sound pressure.
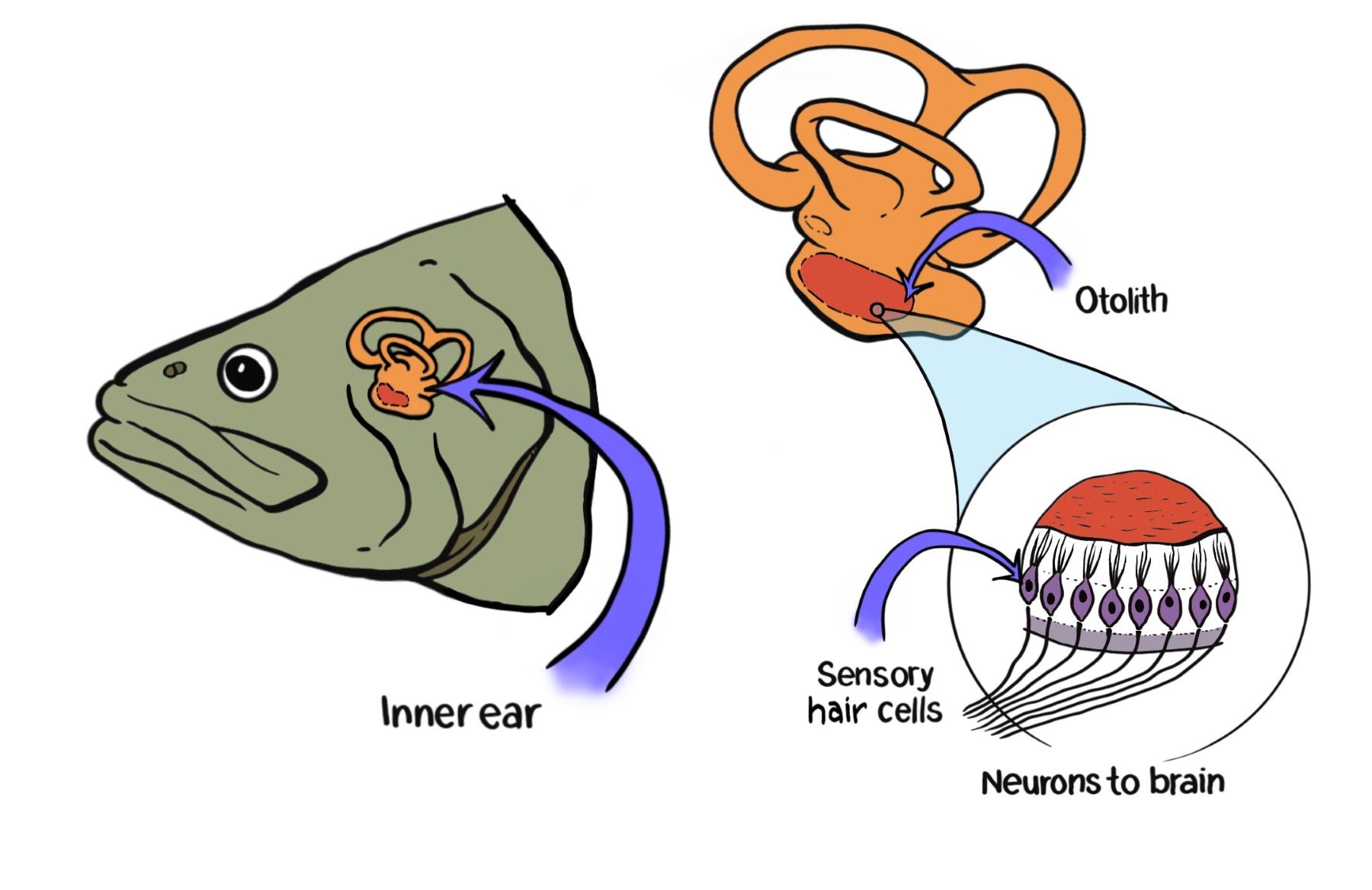
Particle Motion and Marine Energy
Marine energy is a type of ocean energy technology that generates electricity from waves, tides, ocean currents, and free-flowing rivers using many different types of devices. This industry has the potential to significantly contribute to global energy needs and is gaining recognition as a predictable and reliable form of renewable energy. However, before deploying these systems in ocean ecosystems, the marine energy industry and environmental research community are working to understand and proactively mitigate the potential environmental effects of these novel energy converters on fishes and aquatic invertebrates.
Significant effort has gone into measuring sound pressure emissions and the effects associated with offshore energy devices deployed in marine ecosystems. However, since many marine animals use particle motion and substrate vibration, by only measuring sound pressure exposure, there leaves a significant knowledge gap surrounding the effects of noise on a vast number of species.
Moreover, it is not yet well understood whether sound pressure measurements can be converted to accurate estimates of acoustic particle motion in the shallow and bathymetrically complex environments typical of many ocean-based renewable energy sites. This is an active area of research acousticians are working to address.
Like nearly all offshore industries and anthropogenic activities, an environmental stressor of interest for the marine energy community is the potential effects of underwater noise inputs on marine species. To date, sound measurements from the offshore sector have focused on understanding and evaluating the potential impacts of sound pressure on marine mammals. However, without an understanding of particle motion, the picture of underwater noise impacts of marine energy and other anthropogenic activities on aquatic life is incomplete, as fishes and invertebrates make up the greatest portion of marine animal biomass.
Sound pressure is a scalar measurement, meaning it is described by just one value—its amplitude. On the other hand, particle motion is a vector quantity, which includes both the direction and amplitude of the propagating acoustic energy. This means that while a single sound pressure measurement can only tell us how “loud” a recorded sound is, particle motion measurements also contain information about the direction of the sound source.
The primary challenge between sound pressure and particle motion observations is partially due to the difference in access and use of technologies. Hydrophones are sensors that measure underwater acoustic pressure. There are also established methods and tools to collect, process, and analyze acoustic pressure data. Conversely, water-borne particle motion, or vector field measurements, are more complicated (since, for example, they must measure motion on three axes), and there are only a handful of commercially available options for underwater vector sensors. Additionally, particle velocity or acceleration measurements require extra processing to derive bearings of sound sources.
Most importantly, in contrast to knowledge gained from studies of marine mammals’ response to sound pressure, we have almost no baseline for how fishes and invertebrates respond to particle motion, let alone their sensitivity thresholds to these sounds. Understanding the way noise emissions impact fishes or invertebrates requires looking at these issues from the animal’s perspective to understand which signals have the most significant impact on their lives (Popper et al., 2020).
Triton’s Role in Acoustic Particle Motion Research
Triton’s research and development projects, including the Acoustic Particle Motion Project, conduct exploratory research necessary to address complex questions for the marine energy industry. Haxel, who has been studying underwater acoustics related to marine energy for over a decade, investigates ways to improve the characterization of underwater sound emissions and helps inform appropriate international standards for the industry. For several years, Haxel has advocated for more research and attention on particle motion and substrate vibration to holistically address underwater noise effects for all species. To tackle this multidisciplinary work, he has partnered with an experienced team of fish biologists, physicists, engineers, regulators, and acousticians.
Triton’s Acoustic Particle Motion Project began in 2022 with a workshop that convened marine resource management regulators, researchers, engineers, industry subject matter experts, and academic leaders in underwater acoustics and fish physiology—including Arthur N. Popper, PhD. Popper is professor emeritus of biology at the University of Maryland, College Park, and a key partner to Haxel throughout the project.
Popper’s understanding of particle motion, fishes, and invertebrates has come from decades of research and scientific inquiry. At the meeting, he presented to a room full of researchers, regulators, and practitioners to make the case that particle motion was a worthy topic to tackle for the marine energy industry. The 2022 workshop helped ignite meaningful discussions about particle motion research needs to fully address regulatory concerns related to underwater noise impacts.
Following the workshop, Popper, Haxel, and colleagues wrote a perspectives paper from the workshop findings. In this paper, “Marine Energy Converters: Potential Acoustic Effects on Fishes and Aquatic Invertebrates,” the authors provide an analysis of major data gaps in the understanding of acoustic particle motion from marine energy converters and the possible effects of anthropogenic particle motion on aquatic life forms. The publication provided a necessary foundation to help bolster recommendations for research and future efforts.
These conversations piqued the interest of both WPTO and the Bureau of Ocean Energy Management (BOEM), an agency equally dedicated to understanding the impacts of the development of renewable energy systems in U.S. waters. From there, Triton’s Acoustic Particle Motion Project was born.
Shane Guan, PhD from BOEM’s Environmental Studies Program (a co-author in the workshop paper referenced above) has become a key partner in the project with expertise in marine acoustics and substrate vibration. According to Guan, “results from this study would provide valuable knowledge to BOEM for science-informed decision making and environmental impact assessment when planning offshore renewable energy development."
Complex Questions, Complex Approaches
Like most questions involving ocean processes and ecosystems, developing an understanding of the potential environmental effects of acoustic particle motion and substrate vibration is not straightforward.
Researchers can measure particle motion in two ways: estimating particle motion based on sound pressure measurements or using vector sensors that make direct measurements. In deep open water environments, away from the sea surface and ocean bottom, particle motion can be calculated from sound pressure measurements if the water density and underwater speed of sound—two parameters that are easy to measure—are known.
However, as sound propagates in coastal environments, it reflects off the water’s surface, the seafloor, and other objects in the water column like reefs or offshore infrastructure. These “boundary conditions” complicate the relationship between particle motion and sound pressure in shallow or bathymetrically complex environments. Because marine energy is often deployed in shallow waters, research investigating this relationship requires independent measurements of both sound pressure and particle motion to better understand how to best monitor these sound types and gain a better understanding of the potential environmental effects of acoustic emissions for all species.
At the start of the project, Haxel and the Triton team worked with industry partners at JASCO Applied Sciences and at Geospectrum Technologies Inc. to integrate and test a portable, battery-operated instrument package with both a particle motion sensor and a hydrophone. The package includes three-axis accelerometer-based sensors and a reference sound pressure hydrophone to provide comprehensive and independent assessments of both sound pressure and particle motion at a given deployment site. This instrument, called a vector sensor hydrophone (VSH), was field-tested in Sequim Bay, Washington using an acoustic projector. The projector is a calibrated sound source that generates signals at user-specified frequencies and amplitudes.
Through these initial tests, the team is working to validate the ability to quantify particle motion, including the amplitude of the sound and bearing to the source with the VSH. The team also deployed the VSH near an operational small-scale tidal turbine in the tidal channel of Sequim Bay as part of a Testing Expertise and Access for Marine Energy Research Program (TEAMER) test—a collaboration with the University of Washington and Integral Consulting, Inc. Next, the Triton team will take the VSH to the U.S. Navy Hawai’i Wave Energy Test Site to deploy it around operational wave energy converters in a high-energy offshore environment. These deployments will help the team more holistically characterize underwater noise measurements and gather empirical information about coupled particle motion and sound pressure emissions from operational marine energy devices.
While analyzing data collected with the VSH will help the team make recommendations for measuring particle motion at marine energy deployments, questions remain about the physiological and behavioral effects of particle motion emissions from offshore energy activities on animals. To properly address the many uncertainties in this space, the Triton team must go back to the basics.
To recap, here is what we currently know about the effects of particle motion on fishes and invertebrates:
- The auditory system of fishes is sensitive to particle motion, while only a smaller number of fish species can also detect sound pressure.
- Aquatic invertebrates that detect sound are only sensitive to particle motion.
- The auditory system of fishes and aquatic invertebrates has evolved for the detection of particle motion.
- Fishes and invertebrates likely detect and respond to low-frequency stimuli associated with particle motion.
- Invertebrates and fishes that live on, in, or just above the substrate can likely detect substrate vibration.
- Currently limited quantitative data are available to support all the above statements.
Open questions about the effects of particle motion include:
- What frequency range of particle motion do fishes and aquatic invertebrates respond to, and what is their sensitivity to these signals?
- How do fishes and aquatic invertebrates respond behaviorally and physiologically to particle motion and substrate vibration exposures?
- If the animals do respond (behaviorally and/or physiologically) to particle motion and/or substrate vibrations from offshore energy systems, are these responses meaningful for the overall health of the animals and ecosystems?
Taking Particle Motion Research to the Tanks
The project’s next phase, directly supported by both WPTO and BOEM, aims to tackle some of these questions by conducting experiments in tanks and mesocosms—outdoor experimental systems that enable research in the natural environment under controlled conditions. This research approach will dig into the complex questions about how particle motion and substrate vibration may impact fishes and aquatic invertebrates. The project team is exploring behavioral and physiological effects from controlled exposures using projected underwater sounds. Using physiological indicators, such as stress hormone levels, the team will try to quantify a response while correlating any behavioral observations when different acoustic stimuli are presented to the animals. Specifically, Triton will study the effects on species with cultural and commercial significance, beginning with salmon and Dungeness crabs.
To date, scientific inquiry about particle motion on fishes and invertebrates has included sound detection tests on species of shark, stingray (Casper and Mann, 2006), squids (Mooney et al., 2010), and sciaenid fishes (Horodysky et al., 2008) as well as the review of research priorities for the effects of sound and vibration related to offshore wind (Popper et al., 2022). However, even with these studies, there are no quantitative data on animal responses to particle motion levels typical of operational marine energy devices.
The team plans to conduct dose-response experiments in tanks where fishes and crustaceans are exposed to low-frequency sound and substrate vibration using an acoustic projector and an electromagnetic shaker, respectively. The acoustic projector will serve as the sound source and proxy for a marine energy converter, and the magnetic shaker will aim to mimic vibrations in the substrate. Triton is working with acoustic modelers at PNNL to simulate the sound fields in the tanks based on the source location, amplitude, and frequency, which will help inform the experimental setup. During the sound exposure treatment trials, the sound source will simulate a type of sound emission from a tidal turbine. The team will observe animal behavior during the trials and take samples from the animals to measure stress hormones and other physiological markers to understand changes following novel exposure. One of the benefits of conducting tests with animal subjects in tanks, compared with open water tests, is that we can control environmental variables. While this approach may not fully indicate what wild animals might do in their natural habitat, this work is an essential step to understanding levels of particle motion or substrate vibration that lead to behavioral or physiological response and will better inform follow-on research.
Still, these research aspirations are not without their difficulties. For one, the hard walls of tanks reflect sound, making it difficult to achieve the desired sound levels at different frequencies within the tank. Further, if the fishes studied have swim bladders (as do salmon), even the presence of the fishes in the tank can change how the sound propagates. Additionally, substrate vibration in the wild is challenging to realistically reproduce in a tank due to differences in the depth of the sediment and the way the signal propagates through the substrate compared to the ocean habitats. Working with living animals also introduces a variety of variables that must be controlled—like the temperature and salinity of the water. With these and other challenges in mind, the team is currently exploring various experimental setups to conduct the trials and minimize the impacts of these physical and biological factors. For instance, following methods used in previous research for substrate-borne vibration exposure in a tank with hermit crabs (Roberts et al., 2016), the Triton team will employ a similar approach to investigate the sensitivity of Dungeness crabs to varying frequencies and levels of vibration representative of marine energy systems.
While we are still laying the bricks on the path forward in particle motion research, the Triton team is excited to be at the forefront of this investigation. Triton is grateful for the collaborations with scientists at WPTO, BOEM, and the many external subject matter experts making this work possible.
To keep up to date with Triton’s Acoustic Particle Motion project and other research, subscribe to our monthly newsletter here!
Story written by Cailene Gunn and Joe Haxel. Many thanks to Art Popper and Shane Guan for their reviews and contributions to this story.