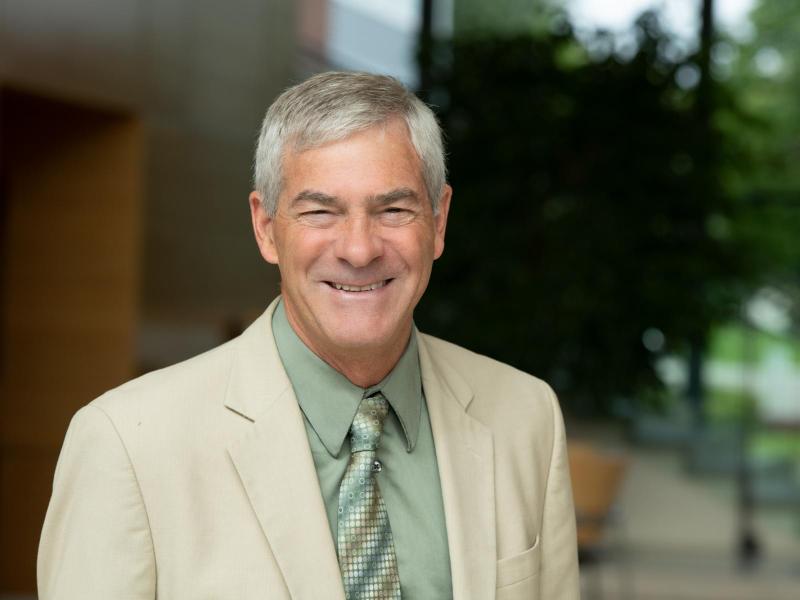
Precision
Materials
by Design
Precision
Materials
by Design
New nuclear and materials science capabilities in the Radiochemical Processing Laboratory (RPL) Radiological Microscopy Suite will benefit research across a number of disciplines. Researchers Edgar Buck (left) and Steven Spurgeon are among those using the 300kV JEOL GrandARM-300F aberration-corrected scanning transmission electron microscope.
(Photo by Andrea Starr | Pacific Northwest National Laboratory)
At PNNL, we are using this combination of theory, computation, and experiments to advance knowledge-driven synthesis of materials for applications in energy, optics, electronics, and quantum information sciences.
Quantum materials synthesis and characterization
Much remains to be learned about the role of material purity in the function of quantum computing devices, starting with synthesis and continuing through to device fabrication. Improvements in quantum gate stability will depend in part upon precision materials characterization that relates structure (angstrom to micron scale) and purity (impurity analysis at the parts per trillion to parts per million scale) to device performance. To this end, PNNL combines expertise in controlled materials synthesis and precision characterization techniques, including:
- Aberration-Corrected Scanning Transmission Electron Microscopy
- Helium/Neon Ion Microscopy/Lithography
- Plasma-Based Focused Ion Beam
- Nano-/Micro-Computed Tomography
- Atom Probe Tomography
- Large Geometry Secondary Ion Mass Spectrometry
- Multi-Collector Inductive-Coupled Plasma Mass Spectrometry.
Understanding atomic-to-mesoscale synthesis
To control the creation of new functional materials, it is necessary to understand the forces that shape them at different scales. Our studies span the continuum from subatomic, to atomic, to molecular, to nanoscale, and beyond. These fundamental studies create foundational knowledge that will allow us to achieve real-time adaptive control over materials synthesis.
For instance, an understanding of superlattice structures formed during nanoparticle self-assembly is important to tailoring superlattice properties. However, key questions remain about how individual nanoparticles behave within emerging superlattice patterns. PNNL researchers are using advanced transmission electron microscopy equipped with direct detection camera capabilities to track individual nanoparticles in real time. Measurements obtained using these tools allow a calculation of the atomic forces competing to drive the assembly process, providing a fundamental understanding of the dynamics involved in the assembly process, and ultimately enabling investigators to control the design of nanoparticle superlattices.
Making better materials atom by atom
Studying the nanostructure of metal alloys atom by atom allowed PNNL researchers to see their alignment and then manipulate it to make the strongest titanium alloy ever developed. The team used electron microscopy to study the metal at nanometer scale and used 3D atom probe tomography to study individual atoms at EMSL, the Environmental Molecular Sciences Laboratory, a DOE Office of Science user facility. This new understanding could lead to the creation of light, high-strength alloys for vehicles and other industrial applications.