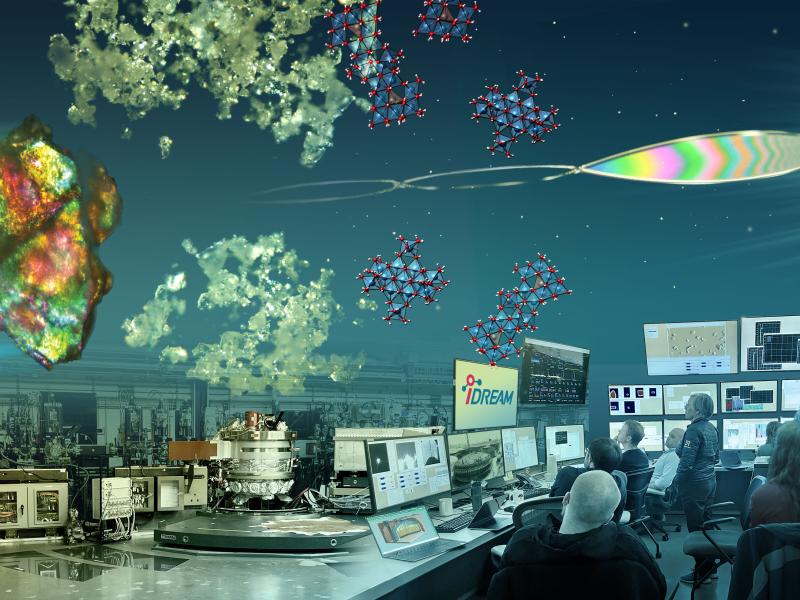
Science of
Interfaces
Science of
Interfaces
Scientists at Pacific Northwest National Laboratory utilize staining procedures for their electron microscopy work. This dendritic crystal formation of tungsten-based negative stain occurred during a staining procedure.
In science, interesting things happen at the edge. In the middle of a block of ice, molecules are locked into a regular pattern and not much is happening. But where ice meets liquid water, or where water meets air, things change. At those edges—what in science are called interfaces—molecules and individual atoms exist in special environments and encounter forces that change their behavior. It’s at these interfaces that opportunity abounds for novel science. And it’s here that PNNL research groups have made strides in revealing previously hidden scientific phenomena that govern material behavior, understanding the root causes of these phenomena in order to control them.
Solvation phenomena at heterointerfaces
Even a substance as ubiquitous as water has secrets still to uncover. The behavior of water drives processes as common and important as rusting and evaporation. But how salt ions behave at the interface of liquid water and air isn't well known. Researchers at PNNL studying that interface discovered that liquid water slows the separation of ions at the interface with air. When surrounded by liquid water, salt ions rearrange water's bonding structure, breaking and forming hydrogen bonds. The insight came from a simulation run on massively parallel computing using resources provided by the Department of Energy’s Office of Science, Basic Energy Sciences. Unlike other simulations, the calculations included thermodynamic and kinetic parameters that had never previously been considered. Their results provide valuable insight into critical interfacial phenomena, enabling controlled materials synthesis.
Transport and bond switching
Interactions at interfaces are different from interactions in the bulk of a material, leading to differences in the way ions, atoms, and molecules move, bind, and react. Researchers at PNNL are trying to control these atomic-scale phenomena by controlling the structure and composition of interfaces. Examples include studying crystal formation on surfaces, how nanoparticles assemble across scales, and the role of water on geochemical nanoparticle dispersions.
Capturing material formation at interfaces
A research team studies the synthesis pathways of thin films and heterostructures of complex oxides with useful functional properties, created by molecular beam epitaxy. They employ a combination of characterization tools, including X-ray photoelectron spectroscopy, scanning transmission electron microscopy, and electron energy loss spectroscopy, aided by artificial intelligence (AI) and machine learning (ML) approaches, to reveal and understand microscopic factors that control the initial stages of materials growth. AI/ML-aided imaging helps analyze metastable phenomena at the interfaces, working toward predictive control to drive desirable synthesis outcomes.
Another team studying atomically-precise interlinked molecular clusters at solid interfaces used ion soft landing of pre-designed building blocks and in situ spectroelectrochemical characterization techniques to understand multiscale interactions in mixed dimensionality systems. Few research institutions possess this array of tools for atomic-level understanding of interfacial phenomena. Harnessing electron and ion transport in such hierarchical systems is poised to enable new types of computing and information storage devices.
Using these distinguishing resources, we are working toward the goal of developing predictable, scalable synthesis of materials for energy and information processing applications.