One Size Does Not Fit All: Environmental Monitoring for Marine Energy
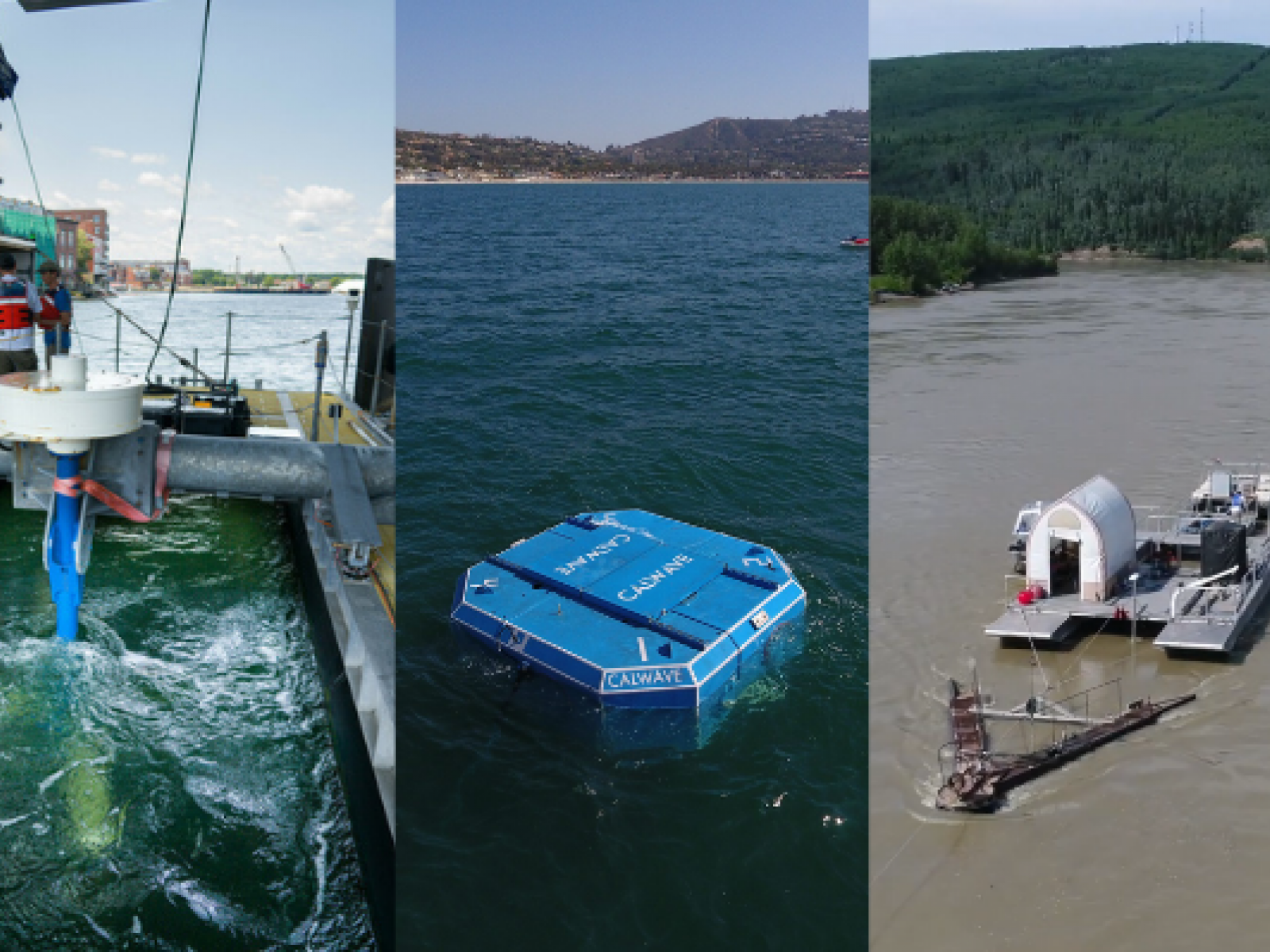
Three marine energy device types, including the Living Bridge Project tidal turbine, the CalWave xWave wave energy converter, and the Tanana River Test Site axial flow riverine turbine.
Jeremy Gasowski | University of New Hampshire (left), CalWave Power Technologies (middle), Amanda Byrd | Alaska Center for Energy and Power (right).
Marine energy devices come in all shapes and sizes and are used to harness the powerful movement of waves, tides, and river currents—even salinity and thermal gradients. Researchers have developed many innovative designs for capturing these valuable renewable resources, from tidal arrays that act like underwater wind farms, to massive bowls in the open ocean that capture overtopping waves and funnel them into electricity. As creative and intriguing as the diversity of marine energy technologies can be, it creates an added challenge for collecting and comparing data that aid in permitting installations. Permitting and regulatory stakeholders are concerned with the potential environmental effects of introducing novel devices into marine and coastal ecosystems, requiring environmental monitoring data to inform decisions.
The reality is, it's complicated. The existence of numerous marine energy devices and environmental settings means there is no one-size-fits-all approach to monitoring. The Department of Energy Water Power Technologies Office’s Triton Initiative is working to find ways to efficiently meet regulatory needs by researching scientifically backed environmental monitoring technologies for studying potential marine energy related impacts. The project's Triton Field Trials (TFiT) explored and tested monitoring technologies for studying potential environmental stressors around various marine energy devices in different settings. As part of TFiT, researchers monitored underwater noise, collision risk, changes in habitat, and electromagnetic fields and then provided recommendations on best practices to the industry (published in the Journal of Marine Science and Engineering special issue). While it is impossible to monitor these stressors around every type of marine energy device and resource, the TFiT team opportunistically sampled relevant stressors at operating devices in a variety of environmental settings.
The first step in researching these systems is understanding how the devices work. Let's look at some of the marine energy devices and learn more about the TFiT project sites.
Wielding the power of waves
Wave energy is one of the most promising renewable resources for the United States. In the Department of Energy's most recent resource assessment, it is estimated that wave energy alone could provide over 30 percent of the energy consumed in the United States in 2019—that could have a considerable impact on the nation's clean energy portfolio.
Waves on the ocean's surface result from wind passing over the water's surface, carrying an immense amount of energy as they move across the sea. They can travel thousands of miles without losing much energy or can combine and get stronger. Wave energy converters (WECs) capture and convert energy from the motion of these waves, which is strongest near the surface and decreases with depth. There are many wave resources worldwide and many locations offshore and nearshore are suitable to harness the energy in different ways.
Point absorbers have a floating structure that moves around a fixed base and captures energy from every direction as water moves near the surface. Surface attenuators are floating devices that often have many connected segments positioned parallel to incoming waves. As the attenuator rides with the swell, internal generators capture the up and down motion and convert the energy to electricity. Overtopping devices are typically located in areas with huge swells. These devices capture water as waves break into a large reservoir, funneling the water into a chamber with a turbine that generates electricity before returning it to the sea. These are only three of many different WEC designs that capture wave energy in many ways, each having unique effects on their surrounding ecosystems. To learn more about different types of WECs, click here.
The Triton Initiative conducted TFiT underwater noise and changes in habitat field tests around the CalWave Power Technologies xWave™ WEC. This novel device dwells just beneath the water's surface and takes advantage of pressure changes as waves pass over the device. These pressure changes create motion in the water column relative to the seafloor. Since the xWave™ is moored and anchored on the seafloor, it can capture this motion and convert it to electricity. The xWave's™ geometry allows for highly efficient conversion of energy, as well as autonomous shutdown, allowing it to go dormant to survive rare but intense storms that can be destructive to infrastructure.
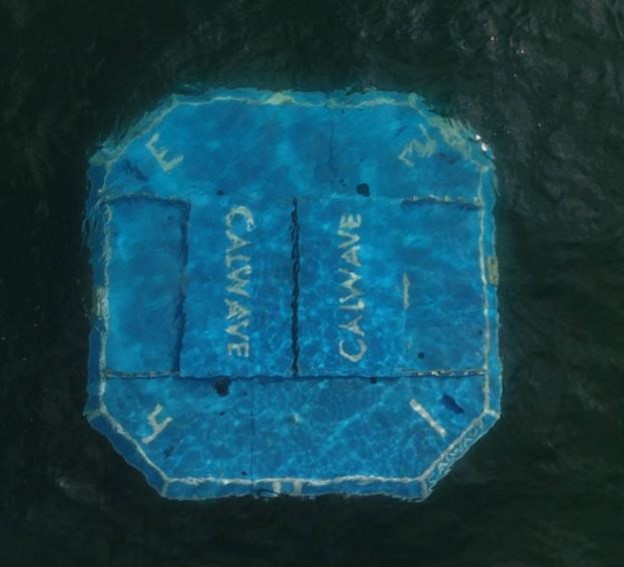
After years of preparation, simulations, tank experiments, and scaled testing, CalWave installed their x1, the first ocean bound version of xWave, off the coast of San Diego. The x1’s maiden voyage is California’s first open-ocean, long duration wave energy pilot project.
In collaboration with Scripps Institution of Oceanography, the bright blue device was towed off the coast of La Jolla, California, for a six-month deployment. The objectives for this demonstration included testing and validating installation procedures, operations, autonomous controls, performance, reliability, and survivability of CalWave’s xWaveTM system in open ocean conditions, providing an opportunity to gather crucial environmental monitoring data. In March 2022, CalWave surpassed the six-month mark of continuous deployment with 99.8 percent operational time. The confidence gained in the stability and reliability of the xWave technology has led CalWave to extend this deployment to gain additional data and incorporate further performance optimizations.
The primary environmental considerations for systems like the xWave™ are underwater noise outputs from the operating device, electromagnetic fields from cables that transport electricity back to shore, and disturbance to local species through collision, entanglement with moorings, or displacement due to its presence. The TFiT underwater noise team has hydrophones deployed around the device to characterize sound emitted from the device in multiple sea states and configurations. Biological evaluations before and during deployment have concluded that based on the technology type, location, and duration of deployment, this project would have no adverse effect on local fish or invertebrate species and it is not likely to adversely affect species of marine mammals, turtles, and seabirds that could potentially visit the project area. In addition, the Triton changes in habitat team also conducted studies on the system to observe possible artificial reef effects, or creation of habitat, on the device's anchors and moorings, which could be a positive ecosystem effect.
While there is still work to be done, this year's xWave™ deployment is a milestone for both the developer and industry in the United States, which has seen few long-term tests on devices of this scale. This is also just the beginning for the xWave architecture. The scale of the current deployment supporting blue economy projects, such as ocean observation, aquaculture, and recharging autonomous underwater vehicles, is growing and a larger scale xWave system is planned to deploy at the PacWave test site in 2024.
Turning tides to electricity
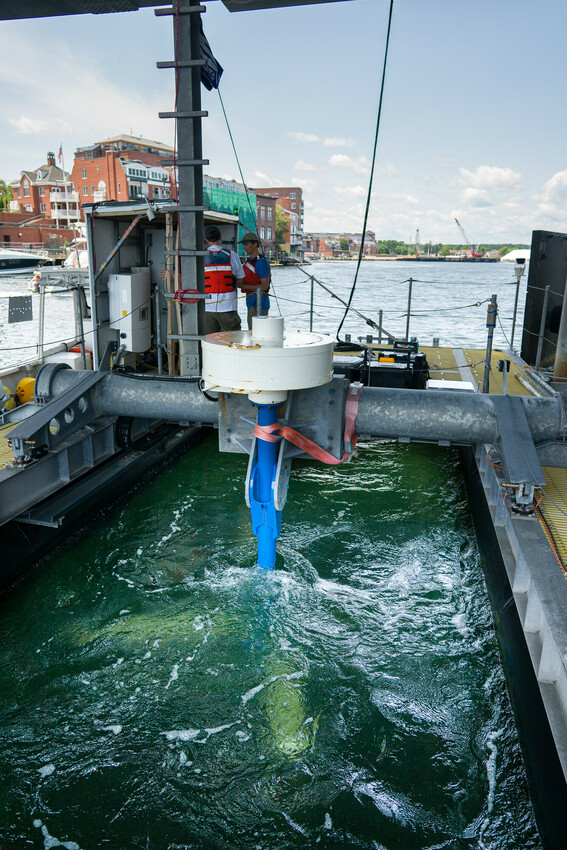
Tidal energy is another marine energy resource getting a lot of attention because it capitalizes on one of the world's most consistent phenomena: tidal cycles. The cyclical rising and falling of the tides that occur on Earth is caused by gravitational pulls from the sun and moon. So long as these celestial bodies exist, tides will rise and fall twice a day, moving massive amounts of water in the process. Certain constricted areas of land, such as inlets and bays, increase the speed at which tides roll in and out of coasts and create powerful resources for energy extraction.
While several types of devices harness tidal power, there are two common types of current energy converters (CEC): axial and cross-flow turbines. Both types work very similarly to wind turbines. Typically, these turbines have multiple blades connected to a shaft, which creates a rotor. As moving water passes over the turbine blades, the motion of the water moves the blades and rotates the shaft, turning the kinetic energy of the water current into mechanical energy. That mechanical energy is then converted to electricity using a generator. Axial flow turbines face the flow, with their axes aligned with the flow direction, and spin as water moves in and out with the tides. They also can have blades that adapt to the direction of the current to make sure the blades capture the most substantial flow, and therefore have the highest energy conversion. Cross-flow turbines are oriented perpendicular to the flow, with blades moving in and out the flow—this means they can spin regardless in which direction the water is flowing. Both types of turbines are often mounted to the seafloor, but they can also be floating or suspended in the water column. They generate electricity both as single devices or as large arrayed systems.
The University of New Hampshire Living Bridge Project uses a vertical axis cross-flow turbine to help power Memorial Bridge in Portsmouth, New Hampshire, and an attached research platform. The Memorial Bridge crosses over a tidal estuary between Portsmouth, New Hampshire and Kittery, Maine, and is equipped with sensors that monitor the bridge's structural performance and traffic patterns and environmental data, such as weather and tidal information. A floating research and turbine deployment platform is connected to the bridge and is equipped with a 3.2-meter diameter commercial cross-flow turbine which powers the sensor network. This single turbine can generate enough electric energy to power the equivalent of approximately two households, given the locally available tidal energy resource.
In collaboration with the University of New Hampshire Center for Ocean Renewable Energy and the Atlantic Marine Energy Center, the TFiT underwater noise and collision risk teams conducted field tests on monitoring equipment at this energetic tidal site.
The main environmental concerns associated with tidal turbines, like the cross-flow turbine at the Memorial Bridge, are collision risk to animals caused by attraction to the device or inability to avoid the device in strong currents, electromagnetic fields emitted from transport cables, and underwater noise generated by the device. To address collision risk, the Triton team deployed an acoustic camera and underwater optical video camera to test how well these instruments detect artificial targets in front of the operational turbine under various conditions. These tests helped assess how well these two underwater observation technologies performed at identifying and observing the targets under multiple conditions in the presence of a moving turbine. Triton also deployed a modified commercial, off-the-shelf drifting hydrophone system to record turbine sounds and ambient acoustic conditions. These tests provided important performance information about the technologies for monitoring turbine-generated sound and contributed valuable data about whether the operating turbine contributes noise levels that could disrupt local marine species.
Read about this TFiT collision risk research the recently published paper “Capabilities of an Acoustic Camera to Inform Fish Collision Risk with Current Energy Converter Turbines.”
Turbines: the riverine kind
The United States marine energy portfolio includes energy naturally harnessed from river currents. The difference between riverine CECs categorized under marine energy and traditional river hydropower is that CECs do not dam or impound water but rather harness power from natural flows. CECs used to harness river currents are similar to tidal turbines but are designed to convert energy from water flowing in only one direction. These turbines can be mounted on the river floor, attached to a floating structure, or be suspended in the water column—much like other marine energy devices. The axial flow turbines commonly used to extract riverine energy typically have two or three blades oriented in the flow direction which are mounted on a shaft to form a rotor. The kinetic motion of a water current catches the blades, turns the rotor, and drives a generator that converts the energy into electricity.
While the theoretical potential of the riverine resource in the United States is only around 2.5 percent of the nation's energy consumption from 2019, according to the Marine Energy in the United States: An Overview of Opportunities report, much of this potential exists in Alaska and the western United States. This resource is particularly beneficial for providing consistent power to small, remote, riverside communities. One such river community is Nenana, Alaska, situated next to the Tanana River.
Nenana is home to the Alaska Center for Energy and Power's Tanana River Test Site (TRTS). This site is a part of the Pacific Marine Energy Center and is used to test riverine power generation devices and characterize the river environment in realistic Alaskan conditions. The TRTS has tested a variety of axial flow, crossflow, and oscillating CEC technologies. The site has a pontoon barge that acts as the infrastructure for deploying and retrieving in-river hydrokinetic turbines and conducting monitoring studies. The barge is versatile and accommodates different turbines that can be lowered into the water column from the floating platform.
The main environmental question associated with hydrokinetic turbine technologies is with fish that swim downstream past the turbine and, in some cases—such as for anadromous species like salmon—swim back upstream after spawning. The turbine can create a potential obstacle for these swimming animals, making it essential to monitor fish populations at riverine test sites and how they behave around moving turbines.
In collaboration with experts at the University of Alaska Fairbanks and the folks at Alaska Center for Energy and Power, Triton conducted TFiT collision risk trials in an environment very different from the Living Bridge. TRTS was the perfect site for conducting riverine field trials because the Tanana River is known for its high flow and turbid waters, making it ideal for testing acoustic monitoring technologies for fish collision research. The team deployed acoustic cameras at this site to observe fish activity in low-visibility, high-energy environments where video cameras are ineffective. University of Alaska Fairbanks fish biologists then fished downstream of the equipment to ground-truth the data outputs and measure accuracy. These murky, challenging conditions are the reality for many rivers suitable for CEC installations, so these tests are valuable for understanding the best tools for the job of monitoring for collision risk.
Diverse devices mean diverse monitoring
Each type of marine energy device is unique and interacts with its specific environment differently. In turn, monitoring must be tailored to site location and the particular specifications, movements, and stressors associated with the individual properties of each device. This is why environmental monitoring research for the marine energy industry is so extensive and complicated. TFiT aimed to address this challenge by conducting field tests around several different marine energy devices and resource types to help provide recommendations on best practices for monitoring specific stressors. However, how monitoring instruments perform will be particular to each location and system. The sharing of baseline and deployment environmental data will help build a robust dataset to help understand the nuances of environmental effects for the many types of devices out there. In addition to collecting and sharing monitoring data, other tools like improved data processing and predictive modeling are needed to increase the comparability and transferability of data for similar project sites.
While advancing rapidly, the marine energy industry is still in its testing and development phase, which means researchers are learning in the process. There is no perfect clean-energy solution. However, with its massive potential, marine energy can provide consistent, carbon-free electricity to communities around the world—from remote river villages to large, coastal urban cities. As the industry progresses, it is the job of researchers to develop and test the tools needed to assess environmental risks associated with this development. Triton plays one role of many working to build a sustainable future for humans and the marine species that will share their habitats with these devices.
Written by Cailene Gunn.
Published: April 20, 2022