Spent Nuclear Fuel Modeling Advances Reactor Deployment
PNNL paper uses SCALE Code System to compare TRISO-fueled reactor designs
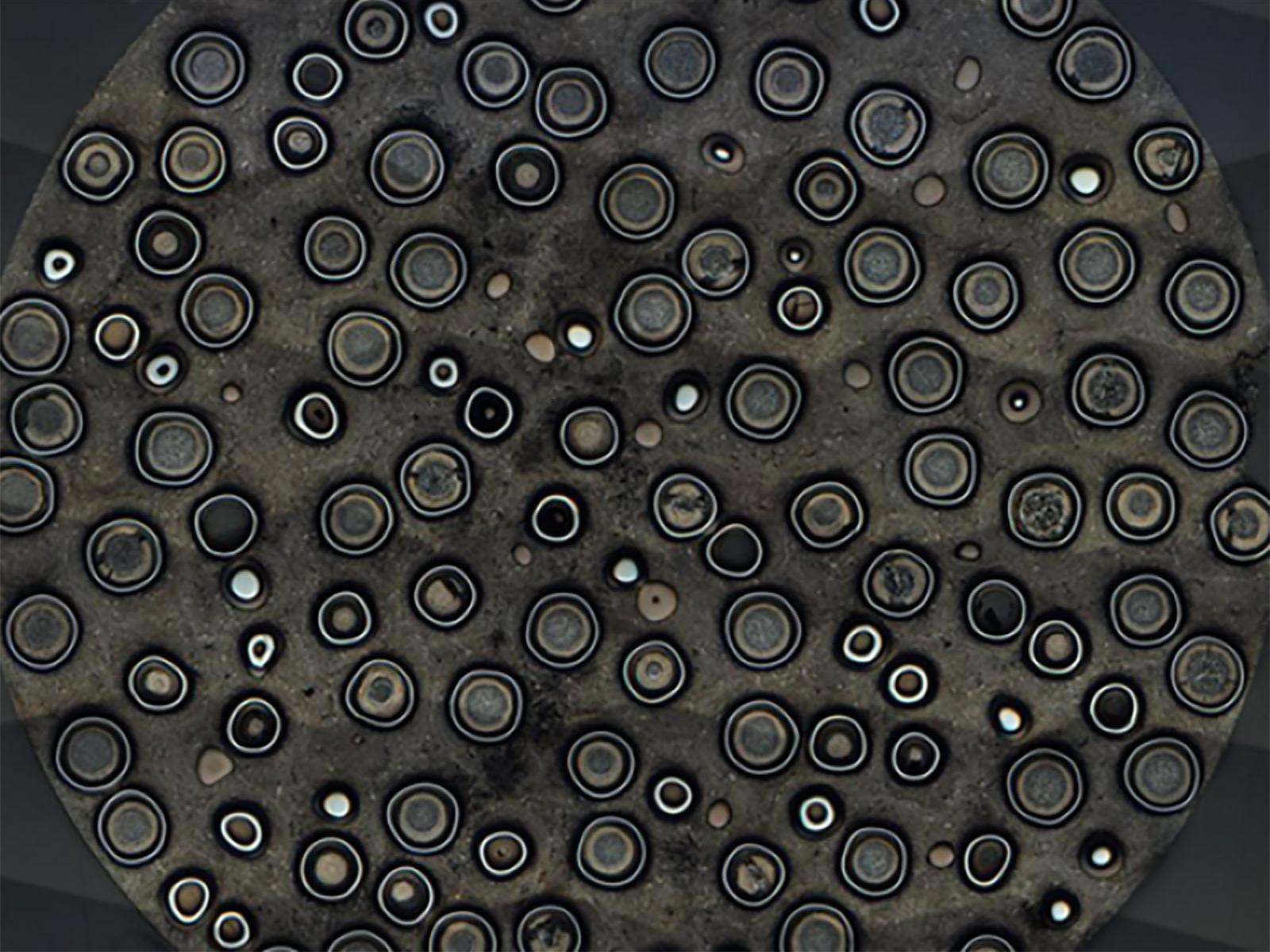
Each TRISO particle acts as its own containment system, thanks to its triple-coated layers. This allows them to retain fission products under all reactor properties.
(Photo: Department of Energy, Office of Nuclear Energy)
With more than 440 commercial nuclear reactors worldwide, including 93 in the United States, nuclear power continues to be one of the largest available sources of reliable, carbon-free electricity.
Nuclear reactors are the efficient engine of a nuclear power plant, responsible for the controlled nuclear chain reactions that ultimately create electricity. Considering the important role of reactors in America’s decarbonized energy future, it follows that advanced reactor designs are receiving renewed interest in industry and government applications.
One important aspect of these advanced reactors is the types of fuel used to power them. Using modeling to understand various fuels’ radioactivity—particularly, how the actual reactor design changes fuels’ properties—lends important insight into dose assessment, fuel-handling operations, and spent nuclear fuel transportation, storage, and disposal.
Newer fuel forms differ from traditional light-water reactor fuels, thus requiring new modeling methods. One of these forms, tri-structural isotopic (TRISO) fuel, consists of several layers of graphite and silicon carbide, which encases a fuel kernel. This fuel has many benefits, such as higher thermal limits and a reduced likelihood of fission product release. Progress in modeling this fuel form will help industry better leverage its benefits.
A Pacific Northwest National Laboratory (PNNL) study led by nuclear engineer Pavlo Ivanusa and published in Nuclear Technology showcases useful methods for analyzing the radioactive properties of spent nuclear fuel discharged from advanced reactors fueled with TRISO fuel.
As outlined in the article, PNNL researchers were able to apply these data in the modeling of various TRISO-fueled reactors and understand how differences in design affect the radioactivity of the fuel after discharge and whether those differences are significant. Ultimately, these processes lend a framework for future analysis and outcomes, especially related to advanced nuclear reactor design.
The Science
PNNL researchers used the SCALE Code System—a widely used modeling and simulation suite for nuclear safety analysis and design—to model, deplete, and compare several different TRISO-fueled reactor designs: a helium-cooled prismatic reactor, a helium-cooled pebble-bed reactor, and a fluoride-lithium-beryllium (FLIBE) molten-salt-cooled pebble-bed reactor.
SCALE offered researchers a system that integrated well with the Transport Rigor Implemented with Time-dependent Operation for Neutronic depletion (TRITON) and Oak Ridge Isotope Generation Code (ORIGEN) modules to model and calculate the depletion and decay of TRISO fuel. All three reactor designs were then analyzed over a range of different fuel enrichments.
The activity of all depleted fuel was then compared at various decay times, and all the TRISO reactor designs were compared to each other. A generic Westinghouse 17x17 fuel assembly was used as a baseline comparison. PNNL researchers then compared the impacts of reactor type, initial fuel enrichment, operating power, and maximum burnup and total radionuclide activities at different timescales relevant to the storage, transportation, and disposal of spent nuclear fuel.
The Impact
Overall, the results showed that short-term activities are dominated by reactors with higher operating powers, and the reactor type, initial fuel enrichment, and maximum burnup are of only secondary importance. By considering analyses of long-term time periods, this work showed that the reactor type and maximum burnup have strong impacts on activities; initial fuel enrichment has a secondary impact, while operating power is inconsequential.
With the ORIGEN results, the FLIBE-cooled pebble-bed reactor was shown to have the highest short-term and long-term activity. Its short-term activity was mainly dependent on the operating power of the reactor before shutdown, consistent with decay heat expectations. As the FLIBE-cooled pebble-bed reactor had the largest specific power, it had the highest total activity. When controlling for operating power, a generic W17x17 light-water reactor fuel had the highest short-term activity.
For long-term time periods—around 100 years—it was shown that maximum burnup and reactor type are the most important factors affecting total activity, with fuel enrichment being of secondary concern and operating power before shutdown having almost no effect. In this situation, the FLIBE-cooled pebble-bed reactor still had the highest activity of all the reactor types analyzed.
These results are useful for future analyses, such as dose assessment and modeling in post-release scenarios; normal fuel-handling operations; and spent nuclear fuel transport, storage, and disposal. Initial results given the effects of various input parameters were also shown, providing a framework to extend the work into other analyses of spent nuclear fuel from advanced reactors, especially those employing TRISO fuel.
Applying these findings to future reactor design ultimately supports the overall advancement of nuclear power for a reliable, safe, and secure energy future.
Summary
This paper demonstrates that it is possible to analyze post-irradiation radionuclide activities and inventories of TRISO reactors using different SCALE modules. Conversely, this paper also showed that analyses using these types of results, such as dose assessment, need to consider the parameters of study and the impacts of reactor type, fuel enrichment, maximum burnup, and operating power. For example, short-term results are dominated by the operating power before shutdown, but long-term results are highly sensitive to reactor type and maximum burnup while being independent of operating power.
These results can be used for initial dose modeling and assessment, or even during initial reactor design. Stewarding this information helps the U.S. Department of Energy and advanced reactor developers answer questions related to new reactor concepts. These reactors, in turn, have the potential to provided carbon-free power as we transition away from carbon-emitting resources like coal and natural gas.
The Research
The PNNL research team members include Pavlo Ivanusa, Philip Jensen, Caitlin A. Condon, and Amoret Bunn. This research was funded by the Laboratory Directed Research and Development program at PNNL. “Comparison of Irradiated TRISO Fuel Radioactivity in Multiple Advanced Reactor Designs” published in the Sept. 2, 2021, online edition of Nuclear Technology (DOI: 10.1080/00295450.2021.1932174).
Published: September 10, 2021
Pavlo Ivanusa, Philip Jensen, Caitlin A. Condon & Amoret L. Bunn (2021) Comparison of Irradiated TRISO Fuel Radioactivity from Multiple Advanced Reactor Designs, Nuclear Technology, DOI: 10.1080/00295450.2021.1932174