Revealing the Effects of Molybdenum Doping on Carbon Dioxide Conversion
The location of molybdenum dopants within catalytic material affects catalysis at a mechanistic level
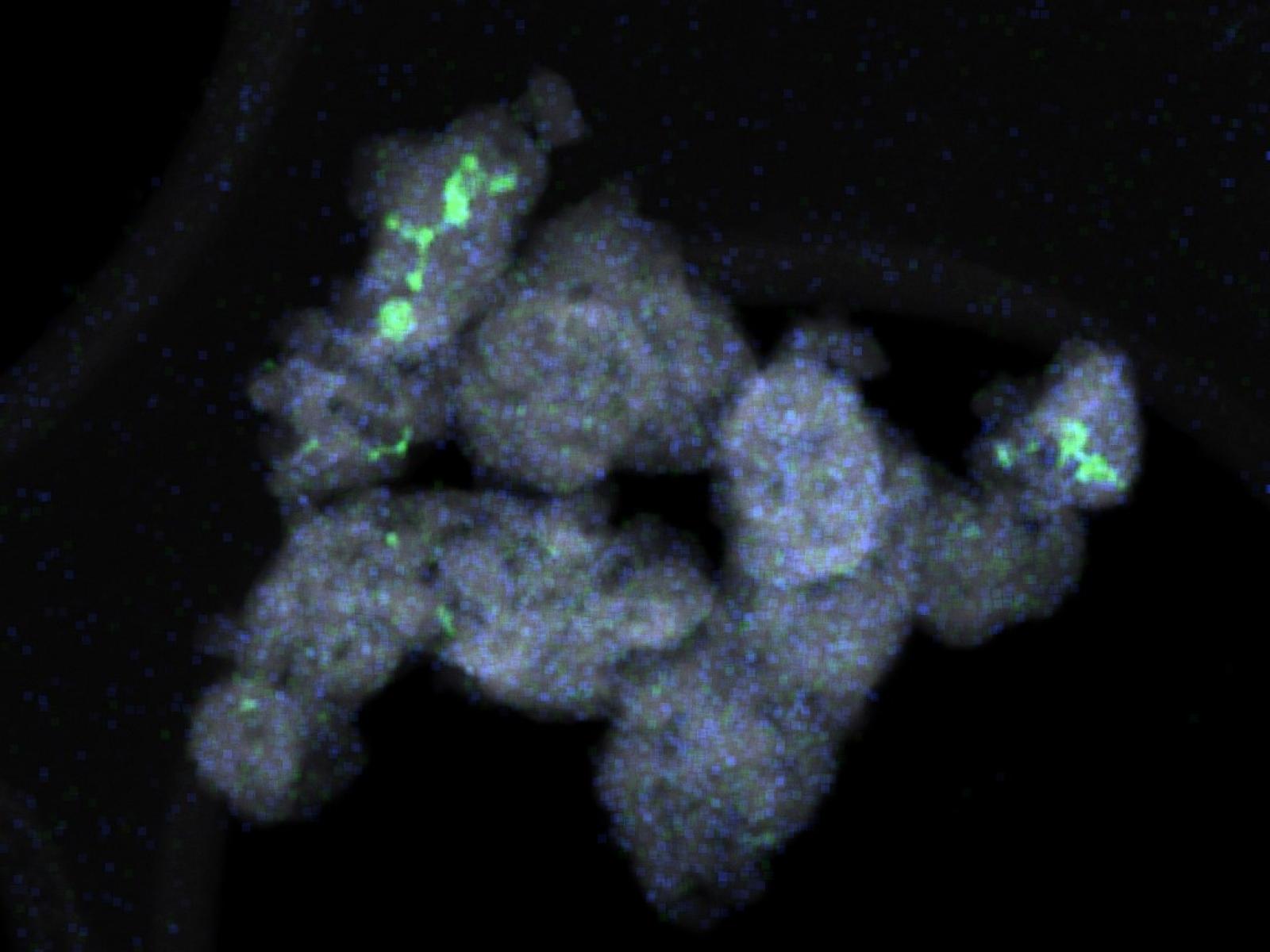
A careful study of a doped catalytic system allowed researchers to identify how the location of the dopants affects the reaction products.
(Image courtesy of Linxiao Chen | Pacific Northwest National Laboratory)
The Science
Metal oxide supported catalysts are a common and stable system used to perform many chemical transformations. In particular, palladium (Pd) on titanium dioxide (TiO2) has been shown to convert carbon dioxide (CO2) into methane and carbon monoxide (CO). Researchers can tune how the system behaves by replacing Ti with differently charged ions, but the exact, molecular-level details remained unknown. New research carefully studied how adding molybdenum (Mo) into TiO2 affected the overall reaction of CO2 on Pd/TiO2. The results show that surface Mo led to the production of more CO than methane by weakening CO bonds with the catalyst surface. Mo within the TiO2 increased the distribution of Pd across the catalyst surface, creating more active sites.
The Impact
Understanding how to create and control catalysts that can turn CO2 into useful products is essential for closing the carbon cycle. While previous work demonstrated that doping TiO2 with other metals affected the system’s reactivity, the underlying mechanisms remained unknown. By specifically identifying how dopant location alters reactivity, this work provides a general approach for catalyst modification. Specifically, this approach can be used to create more efficient and more selective catalysts for CO2 conversion.
Summary
Doping TiO2 supports with differently charged ions is a known, viable strategy for fine-tuning active sites on metal catalysts. However, the roles of different dopant species and the origins of their effects have not been clearly described. This work focused on obtaining this fundamental understanding missing in the literature, using CO2 hydrogenation over Pd/TiO2 catalysts as a model system.
Researchers synthesized TiO2 with Mo dopants in the bulk or on the surface of the material by adding Mo either during synthesis or through post-synthesis impregnation, respectively. Pd nanoparticles supported on surface-Mo-doped TiO2 exhibit decreased selectivity toward methane during CO2 hydrogenation. Combined spectroscopic studies revealed that the presence of highly reducible molybdenum-oxygen clusters near Pd increases the overall reducibility of the support. This leads to higher electron density on Pd particles under reducing reaction conditions, weakening the adsorption of CO on Pd sites and preventing its further hydrogenation to methane. On the other hand, Pd nanoparticles supported on bulk-Mo-doped TiO2 show a higher CO production rate. Microscopy analysis revealed that defects created by bulk Mo substitution of Ti leads to more dispersed Pd, generating more of the Pd-TiO2 interfacial sites responsible for CO production. This work clearly differentiated the role of surface and bulk Mo dopants, revealing the underlying mechanisms behind their effects on Pd/TiO2 catalysts. The results corrected the literature misunderstanding that these dopants affect the performance of the catalysts by altering the band gap of TiO2 by showing that enhanced surface reducibility causes the observed effects.
PNNL Contact
Janos Szanyi, Pacific Northwest National Laboratory, Janos.Szanyi@pnnl.gov
Funding
This work was supported by the Department of Energy (DOE), Office of Science, Basic Energy Sciences program, Division of Chemical Sciences, Geosciences and Biosciences, and was carried out at Pacific Northwest National Laboratory. This research used resources from the Advanced Photon Source, an Office of Science user facility operated for the DOE Office of Science by Argonne National Laboratory, and from EMSL, the Environmental Molecular Sciences Laboratory, also an Office of Science user facility.
Published: October 28, 2022
Chen, L., L. Kovarik, D. Meira, J. Szanyi. 2022. ACS Catal., 12, 13492-13500. [DOI: 10.1021/acscatal.2c03181]