Catalysis
Introduction to Catalysis
Catalysis touches everyone’s life from the catalytic converter in your vehicle, to how you clean your contact lenses, to creating your plastic water bottle. Catalysts make it easier for a chemical reaction to occur. In a chemical reaction, starting chemicals, called reactants, are transformed into different chemicals with new properties, called products. Over the course of the reaction, reactants are consumed and used to make products. An example of a chemical reaction is the rusting of iron. In this reaction, iron and oxygen combine to form iron oxide rust.
Each reaction occurs at a certain rate, which can be altered by adding a material called a catalyst. The catalyst is neither a reactant nor a product; it does not get consumed during the reaction. Changing the rate of a reaction through the addition of a catalyst is called catalysis.
Catalysts work by lowering the activation energy of a reaction—the amount of energy needed for the reaction to proceed. For example, a catalyst may bring two reactants closer together or may stabilize a transition state. Lowering the activation energy allows the reaction to occur more often at a given temperature, increasing the rate of the reaction.
Catalysis can be generally defined into three broad categories: homogeneous catalysis, heterogeneous catalysis, and biocatalysis. In homogeneous catalysis, both the reactants and the catalyst are in solution with either an aqueous or organic solvent. For example, acids can act as catalysts, such as in the hydrolysis of esters. The high homogeneity of these mixtures allows for high reactivity, though separating the mixtures into their respective components may be challenging. Homogeneous catalysis may also be referred to as molecular catalysis.
In contrast, the catalysts of heterogenous catalysis are in a different state than either the products or reactants. This type of catalysis is used for many industrial processes, with gaseous and liquid reactants being passed over a solid catalyst, because of the ease with which the products can be separated and the catalyst can be recovered. Zeolite catalysts are commonly used heterogeneous catalysts. They form solid cage-like structures that gas or solution phase reactants can pass through and be transformed into products.
Biocatalysts are a special class of proteins, called enzymes, or nucleic acids, called ribozymes, that catalyze biological reactions. These can be produced naturally by cells or can be engineered to catalyze new reactions. Advances in molecular biology revolutionized the use of biological catalysts for industrial applications.
The History of Catalysis
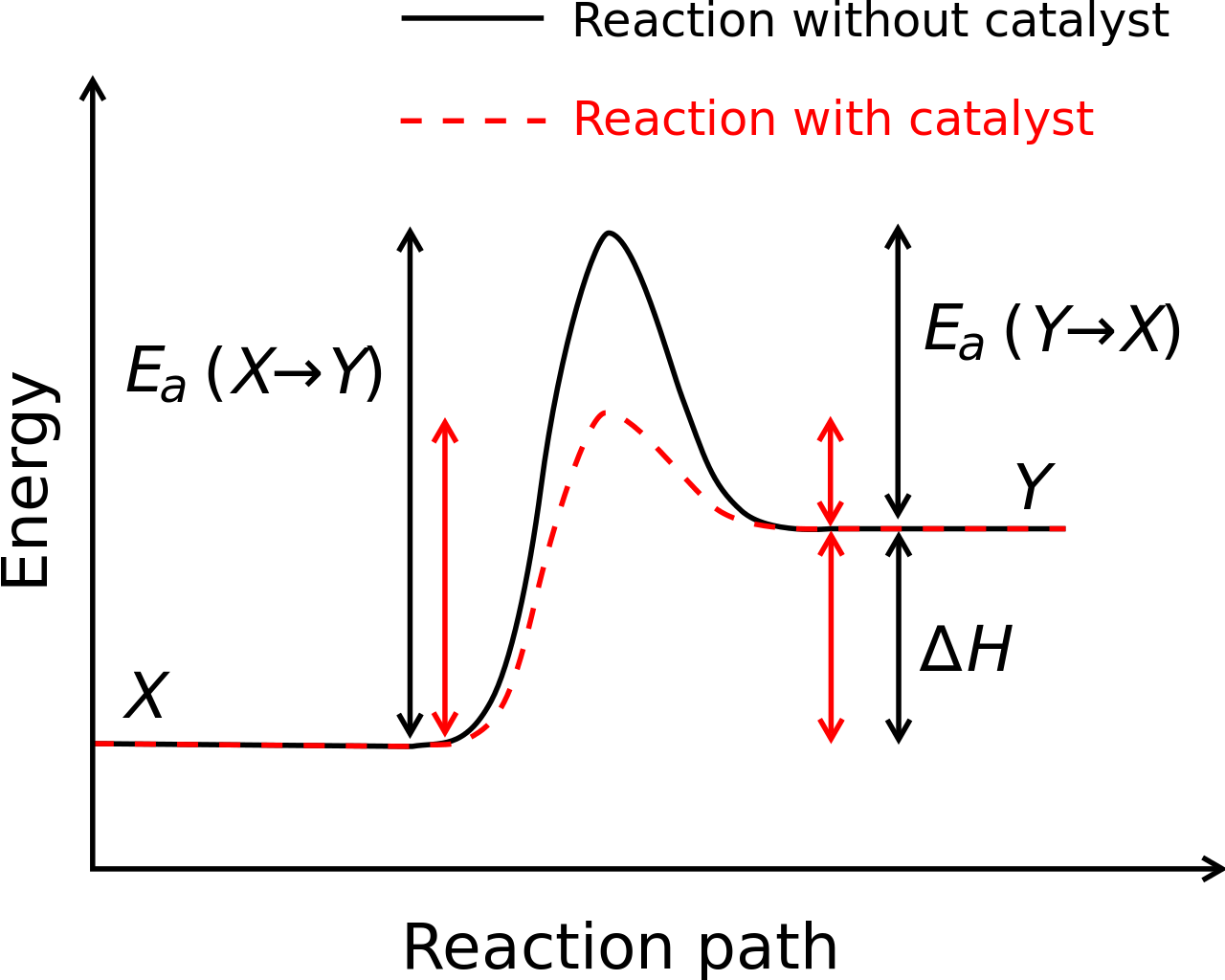
Though catalysts have been used by humans since ancient times, such as to produce alcohol through the fermentation of sugars, the systematic study of catalysis did not begin until the 1700s. In the late 1700s and early 1800s, Elizabeth Fulhame provided a theoretical foundation for the study of catalysts. In 1835, the word “catalysis” was coined by chemist Jöns Jakob Berzelius. Throughout the eighteenth and nineteenth centuries, experiments involving various metals provided evidence that these materials could be both selective and specific catalysts. The work of Paul Sabatier in the late 1800s and early 1900s led to the discovery of many metal catalysts, particularly nickel and platinum group metals. These remain some of the most important catalysts for industrial applications to date for a variety of applications such as crude oil refining and reducing car emissions.
Importance of Catalysis
Catalysts have many industrial uses, from the production of food products, biofuels, pharmaceuticals, plastics, and chemicals. Most commercially produced chemicals are made with the help of catalysts. Some notable reactions enhanced by catalysis include production of sulfuric acid used for rust removal as well as a precursor for phosphoric acid, synthesis of ammonia, petroleum refining, and limiting of vehicle emissions. Because of how integral catalysis is to many industrial processes, scientific research to improve catalysis will benefit many areas of our lives.
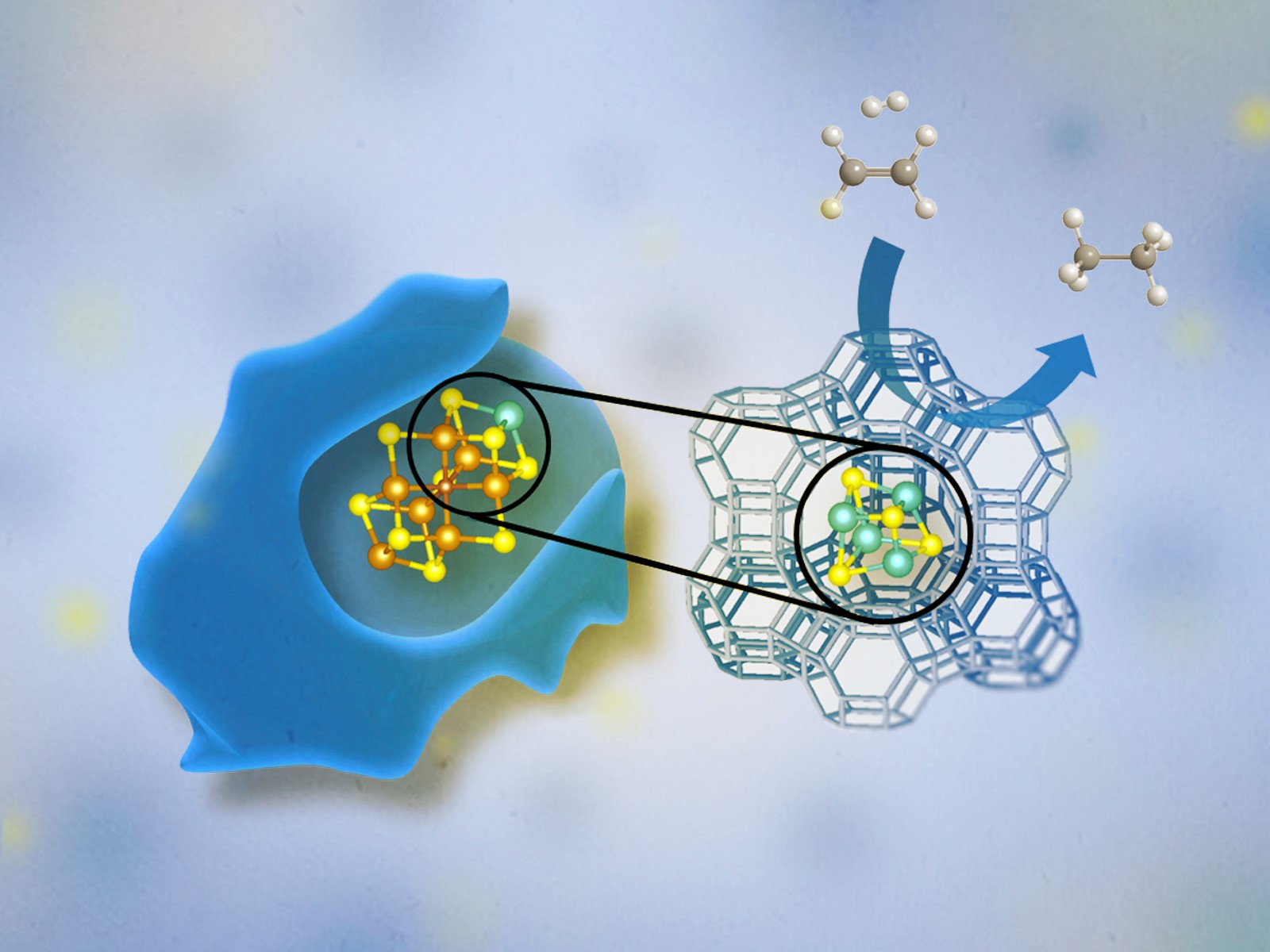
Benefits and Limitations of Catalysis
Each type of catalysis has its own strengths and limitations. Homogeneous catalysis is highly reactive and selective, but separation of products from the catalyst remains challenging due to the homogeneity of the mixture. Heterogeneous catalysts are less reactive and selective, but the products are relatively easy to separate from the catalyst. Biocatalysts can be highly selective but typically have shorter lifetimes than other types of catalysts.
Catalysis for a Better Tomorrow
Catalysts will play a pivotal role in achieving a greener future. They can help produce value-added chemicals for renewable energy, capture carbon dioxide from the air, and provide clean water, among other things. This will help our nation achieve its energy and sustainability goals.
Catalysis at PNNL
Pacific Northwest National Laboratory (PNNL) researchers address some of the grandest challenges in catalysis science through avenues such as bio-inspired design, modeling and simulation, and cost-saving reaction pathways.
New principles of bio-inspired design and synthesis will accelerate the development of catalysts that have increased rates for target reactions under ambient, or near ambient, conditions. The challenge here is to harness the elegant methods of enzymes, which catalyze reactions at low temperature and pressure, and replicate these methods in man-made systems. New approaches to modeling and simulation, synthesis and characterization, will allow precise control of multidimensional catalytic structures and molecular orientation in solution as they interact with reactants. Devising predictive methods to conduct in-silico reactions first will reduce energy and labor costs and have a much smaller environmental impact. And, finding new reaction pathways will target abundant electrons to convert inexpensive substrates.
In the catalysis arena, PNNL has a strong foundation of discovery science. Its Center for Molecular Electrocatalysis (CME) is a U.S. Department of Energy (DOE) Energy Frontier Research Center established by the Basic Energy Sciences program.
PNNL researchers there, along with their nationwide partners, are driven to understand molecular electrocatalysts in fuels—fuels that will efficiently convert electrical energy into chemical bonds, or chemical energy into electrical energy. By bringing together creative, multidisciplinary teams, CME is taking on the toughest challenges in energy technology.
Researchers at CME use powerful tools for characterizing, understanding, modeling, and manipulating matter at scales ranging from the atomic to the macroscopic. For additional tools and opportunities, researchers collaborate with colleagues at the Environmental Molecular Sciences Laboratory (EMSL), a DOE user facility located on the PNNL campus. EMSL houses unique, specialized, and powerful instruments to conduct modeling, simulation, and characterization. For example, PNNL researchers utilized advanced microscopy techniques to reveal the catalytic activity of gold nanoparticles—one of DOE’s basic research needs in synthesis sciences.
In the Institute for Integrated Catalysis (IIC), PNNL combines more than 120 in-house scientists and engineers with experts from universities, industry, and other national laboratories. They collaboratively explore and develop the chemistry and technology of catalyzed processes that will enable a carbon-neutral future. IIC researchers are focused on removing oxygen and adding hydrogen to carbon feedstocks, as well as removing heteroatoms. The researchers search for ways to store electrical energy in hydrogen–hydrogen, carbon–hydrogen, or nitrogen–hydrogen bonds. They also manipulate carbon–carbon and carbon–heteroatom bonds and look for ways to optimize exhaust catalysis.
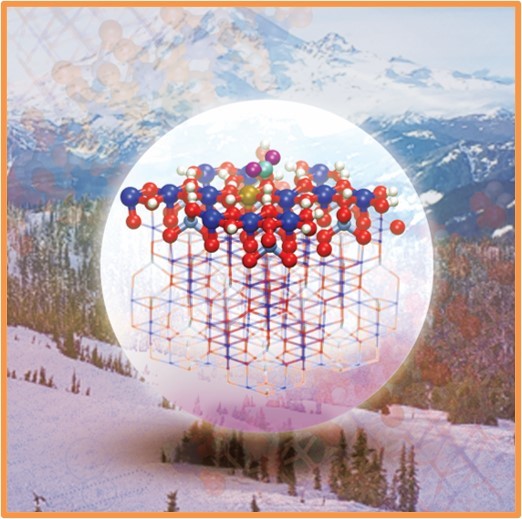
Catalysis is also supported by major in-house investments. The PNNL objective on reinventing chemical catalysts and catalytic processes, for example, supports research to harness catalysis in order to create fuels from cheap, abundant domestic materials. PNNL researchers draw on the attributes of both natural and synthetic systems to develop catalysts that work at energy-saving low temperatures. They also tailor catalysts for safe and cost-effective applications that produce no unwanted byproducts.